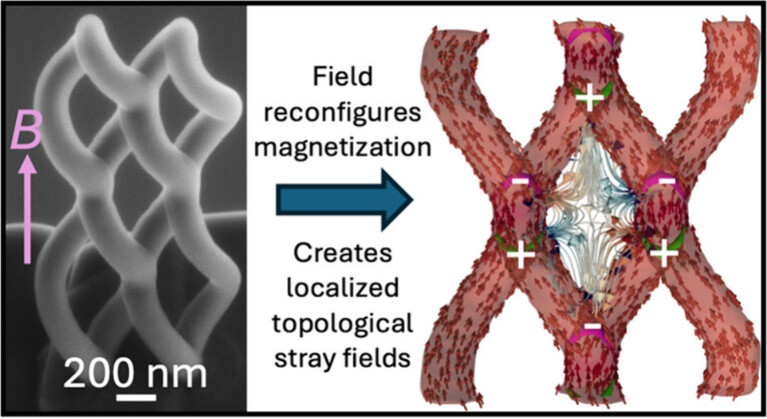
Three-dimensional (3D) magnetic nanostructures are an emerging platform capable of creating complex topological magnetic fields. The control of localized nanoscale magnetic fields is seen to be of importance for diverse areas from bioapplications such as drug delivery, to particle trapping and controlling Majorana Fermions for quantum computing. Three-dimensional geometric confinement and proximity can create tailor-made spin textures not possible in two dimensions. The control of magnetization afforded here can allow the formation of unique stray field textures. Here, we report the creation of reconfigurable 3D topological magnetic field textures induced by an interwoven 3D nanostructure and applied field protocol. These field textures emerge due to distinct DWs formed in this structure and lead to the creation of an antivortex field, a hexapole cusp and a 3D skyrmion field tube of mixed chirality. Our results therefore show a key step toward the design and control of topological magnetic fields on the nanoscale.
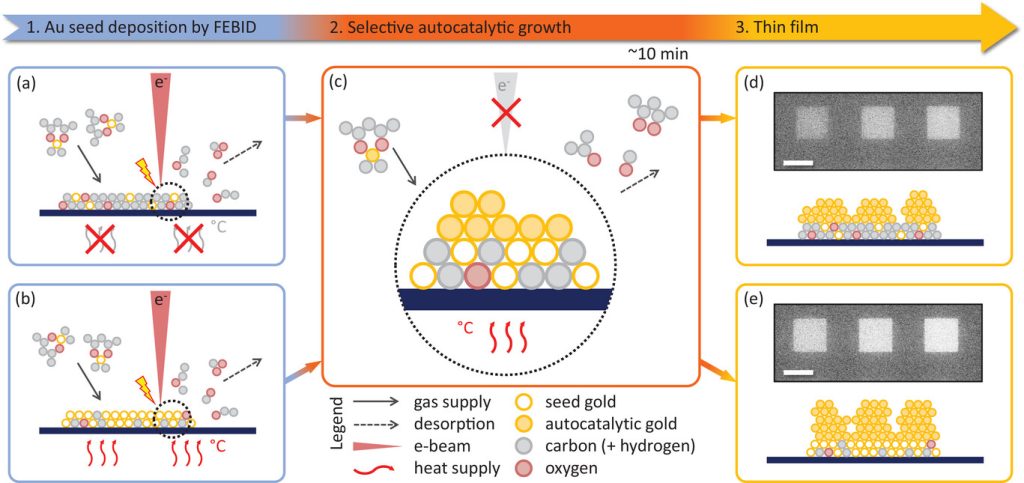
Chemical vapor deposition (CVD) is an established method for producing high-purity thin films, but it typically necessitates the pre- and post-processing using a mask to produce structures. This study presents a novel maskless patterning technique that enables area-selective CVD of gold. A focused electron beam is used to decompose the metal–organic precursor Au(acac)Me2 locally, thereby creating an autocatalytically active seed layer for subsequent CVD with the same precursor. The procedure can be included in the same CVD process without the need for clean room lithographic processing. Moreover, it operates at low temperatures of 80 °C, over 200 K lower than standard CVD temperatures for this precursor, reducing thermal load on the specimen. Given that electron beam seeding operates on any even moderately conductive surface, the process does not constrain device design. This is demonstrated by the example of vertical nanostructures with high aspect ratios of ≈40:1 and more. Written using a focused electron beam and the same precursor, these nanopillars exhibit catalytically active nuclei on their surface. Furthermore, by using the onset of the autocatalytic CVD growth, for the first time the local temperature increase caused by the writing of nanostructures with an electron beam can be precisely determined.
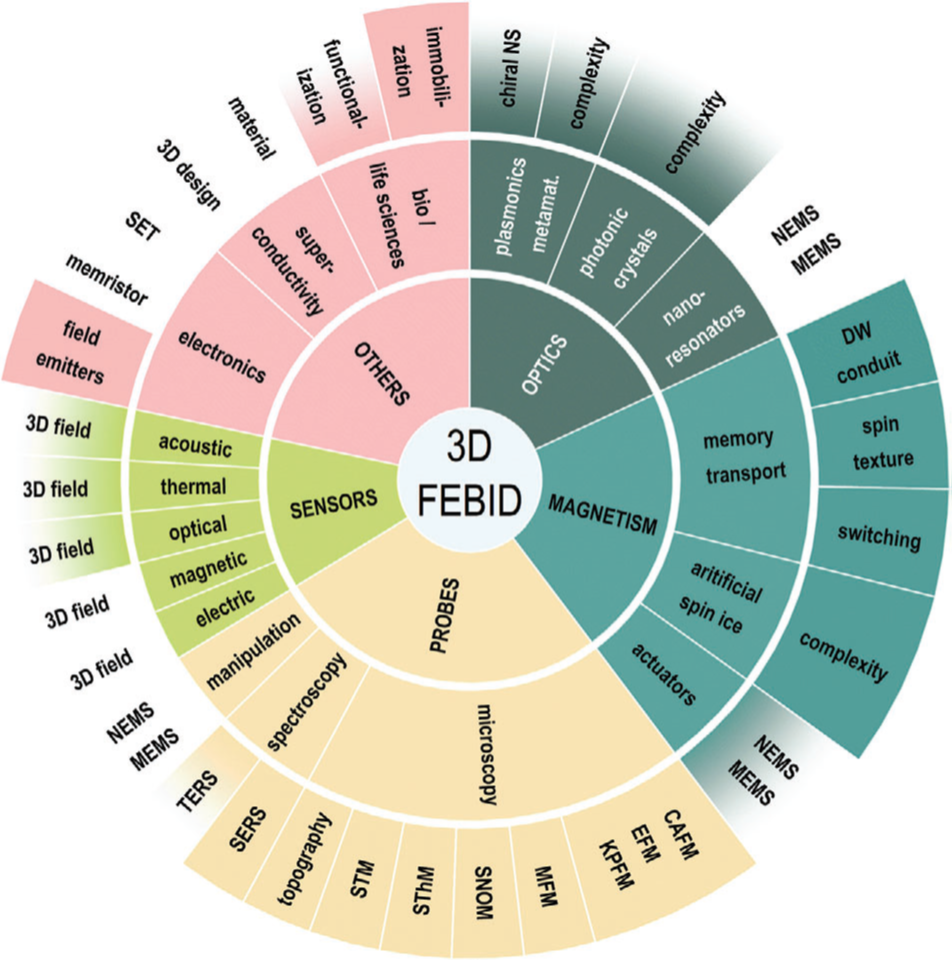
Following Moore`s law, the performance of devices should double within a two-year span, which can either be done by reducing the size of the individual components or adapting the working mechanism. In most cases the same mantra applies – pushing the size while keeping the quality. As manufacturing techniques are approaching the atomic scale, however, miniaturization reaches its intrinsic limit, shifting focus toward higher-dimensional architectures realized by stacking planar layers or fabricating true, free-standing nanostructures. A mask-less, additive manufacturing technique with the capability to produce such 3D structures at the nanoscale is called Focused Electron Beam Induced Deposition (FEBID). Applying a focused electron beam in a conventional scanning electron microscope together with a gaseous metal-precursor gives access to a vast library of complex, 3D nanostructures, which exhibit feature sizes down to the 10 nm-range and can be printed in a single step on most substrates. This review aims at introducing the technique to a broader scientific community, breaking down the most important processing routes and highlighting disciplines explored so far ranging from nanooptics and nanomagnetism, over scanning probes and field emitters to sensing and particle trapping. Finally, a future roadmap of the technique is discussed, and prospective research focus identified.